Battery Classifications and Chemistries
Two Major Battery Classifications– Primary and Secondary Batteries
Batteries can be broadly classified into two categories– primary and secondary batteries. The key distinction lies in the rechargeability of secondary batteries, as opposed to primary batteries, which cannot be recharged. The reactions in primary batteries cannot be easily reversed. As such, when the battery electrodes are used up, they cannot be reverted back to their original state even when an external voltage is applied. On the contrary, secondary batteries can be recharged and used again by applying external voltage. Typically, primary batteries have higher specific energy (in W⋅h⋅kg–1) and power (in W⋅kg–1) than secondary batteries. Side note: specific energy is the energy capacity of the battery per unit battery weight, whereas specific power is the highest power that the battery can produce in a short period of time per unit battery weight.
Feature | Primary Batteries | Secondary Batteries |
Rechargeability | Not rechargeable | Rechargeable |
Typical Chemistry | Alkaline, Zinc–Carbon, Lithium | Nickel–Cadmium (NiCd), Nickel–Metal Hydride (NiMH), Lithium–Ion (Li-ion) |
Usage | Single-use, disposable | Reusable, can be recharged multiple times |
Initial Cost | Generally lower | Higher initial cost, offset by reuse |
Energy Density | Lower energy density compared to some secondary batteries | Higher energy density in some cases |
Environmental Impact | Can contribute to e-waste | Better environmental profile if properly recycled |
Common Applications | Remote controls, flashlights, toys, certain medical devices | Laptops, smartphones, electric vehicles, cameras, power tools, cordless phones, portable audio devices, e-cigarettes, medical devices, uninterruptible power supplies (UPS), renewable energy storage |
Within these two major categories, batteries can be further classified according to their
- Construction, chemistry, or chemical composition,
- Specific energy and power,
- C-ratings, and
- Discharging and charging properties.
Battery Chemistries
Batteries can be classified according to their chemistry or specific electrochemical composition, which heavily dictates the reactions that will occur within the cells to convert chemical to electrical energy. Battery chemistry tells the electrode and electrolyte materials to be used for the battery construction. It influences the electrochemical performance, energy density, operating life, and applicability of the battery for different applications.
Primary Battery Chemistries
Primary batteries are “dry cells”. They are called as such because they contain little to no liquid electrolyte. Again, these batteries cannot be recharged, thus they are often referred to as “one-cycle” batteries.
Primary batteries come in three major chemistries: (1) zinc–carbon and (2) alkaline zinc–manganese, and (3) lithium (or lithium-metal) battery.
Zinc–carbon batteries is among the earliest commercially available primary cells. It is composed of a solid, high-purity zinc anode (99.99%). The cathode is a mixture of NH4Cl solution (10%), MnO2 (60%), carbon black (20%), and water (10%). The separator is a carbon rod, which aids in the transport of electrons and retention of moisture within the cell all while avoiding the contact between the anode and the cathode. The electrolyte is composed of ZnCl2 and NH4Cl. Zinc–carbon batteries can deliver 1.5 V. The overall reaction in zinc–carbon batteries is
2MnO2 (s) + 2 NH4Cl (aq) + Zn (s) ⟶ Zn2+ (aq) + Mn2O3 (s) + 2 NH3 (aq) + H2O (l) + 2 Cl− (aq)
Zinc–manganese batteries are composed of manganese dioxide positive electrode, zinc negative electrode, and ammonium chloride electrolyte. They have the characteristics of heavy load, high current, strong continuous discharge ability, stable working voltage, excellent leak-proof performance, long storage time, and good low-temperature performance. In this battery chemistry, chemical energy is converted via the reduction of the MnO2 cathode and oxidation of the Zn anode, as in the reactions:
Anode Zn + 2 OH– → ZnO + H2O + 2 e–
Cathode 2 MnO2 + 2 H2O + 2 e– → 2 MnOOH + 2 OH–
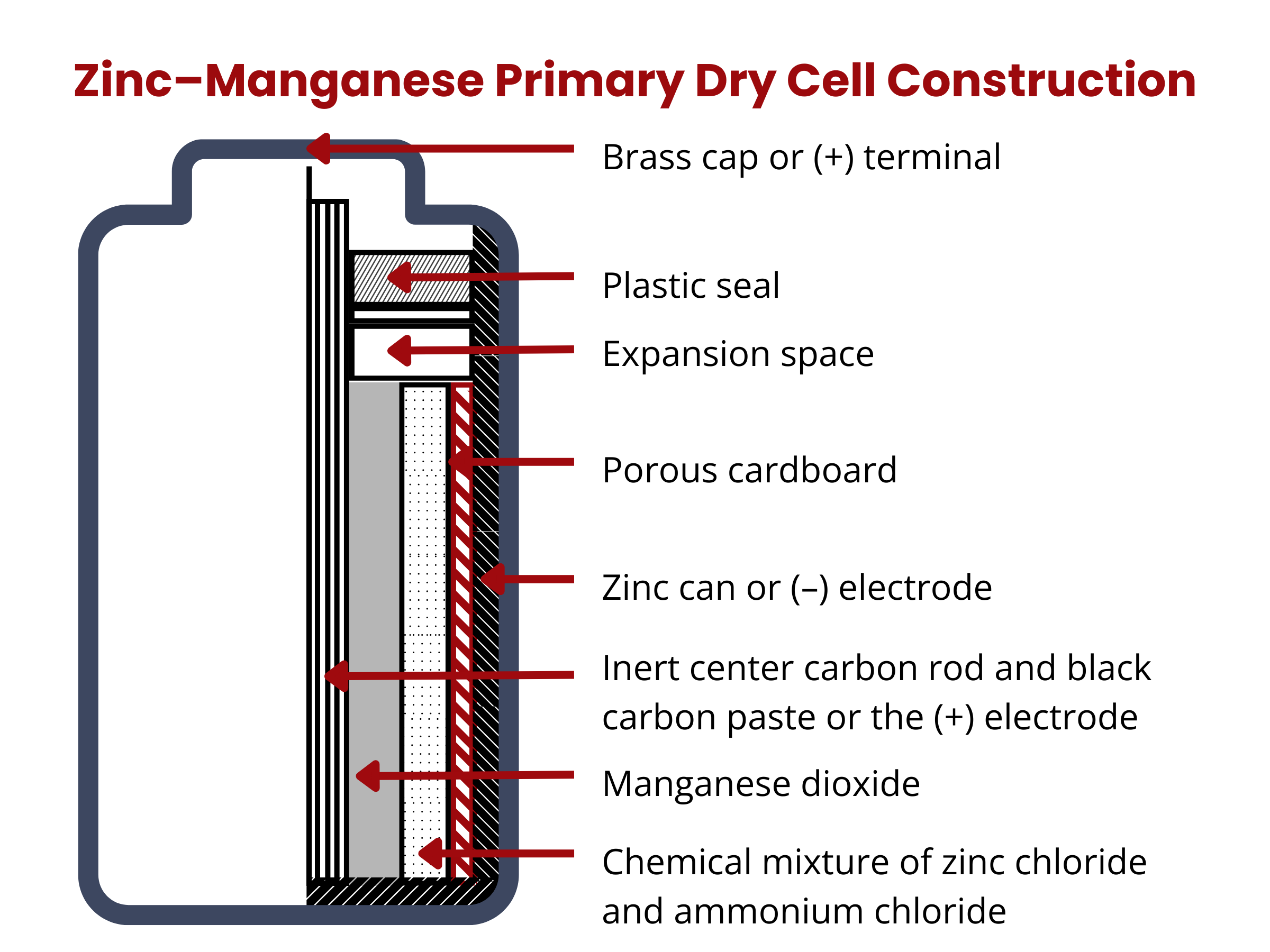
Zinc–manganese batteries were developed to address the issues encountered in zinc–carbon batteries, including their limited lifespan and relatively low energy density. An alkaline battery is capable of providing approximately three to five times the energy output compared to a zinc-carbon dry cell of equivalent size.
Secondary Battery Chemistries
Secondary battery chemistries, distinct from primary batteries, are rechargeable systems where the electrochemical reactions are reversible. Unlike primary batteries that are typically single-use, secondary batteries, such as lithium-ion and nickel-metal hydride, allow for repeated charging and discharging cycles. The key differentiator lies in the ability of secondary batteries to be replenished with electrical energy, making them suitable for applications requiring long-term use and energy storage. The reversible nature of the electrochemical processes in secondary batteries involves the movement of ions between the positive and negative electrodes during both charging and discharging, enabling a sustainable and rechargeable power source for various devices and systems.
Reactions involved in Secondary Battery Chemistries
Battery Chemistry | Anode Reaction | Cathode Reaction | Ions Involved |
---|---|---|---|
Lithium-ion | LiC6 → Li+ + e- | LiCoO2 + Li+ + e- → Li2CoO2 | Lithium ions (Li+) |
Nickel-Metal Hydride | M + H2O → MOH + H+ + e- | NiOOH + H+ + e- → Ni(OH)2 + H2O | Hydrogen ions (H+) |
Lead Acid | Pb → PbSO4 + 2e- | PbO2 + 4 H+ + SO42- + 2e- → PbSO4 + 2 H2O | Hydrogen ions (H+), Sulfate ions (SO42-) |
Lithium Iron Phosphate (LiFePO4) | LiFePO4 → Li+ + FePO4 + e- | FePO4 + Li+ + e- → LiFePO4 | Lithium ions (Li+), Iron ions (Fe3+, Fe2+) |
Lithium Polymer (Li-Po) | Similar to Lithium-ion with different physical structure | Similar to Lithium-ion with different physical structure | Lithium ions (Li+) |
How do batteries work?
Inside the battery, chemical reactions, namely oxidation and reduction, occur at the electrodes. During discharging, oxidation occurs at the anode, releasing electrons that travel through the external circuitry to the cathode. At the cathode, these electrons are used up during the reduction reaction. At the same time, ions move through the electrolyte. This movement maintains charge neutrality within the battery. The flow of electron through the external circuit creates an electric current, therefore discharging the battery.
Primary batteries can only be discharged. Once their chemical reactions are depleted or reach a point where they cannot sustain the necessary voltage, the battery is considered "dead" and cannot be recharged. The electrochemical reactions that generate electrical energy in primary batteries are typically not reversible, distinguishing them from secondary batteries, which are rechargeable and allow for repeated charging and discharging cycles.
To charge secondary batteries, an external voltage is applied. This applied voltage reverses the electrochemical processes. The electrons are forced to move from the cathode to the anode, essentially restoring the reactants and allowing the battery to be reused. As long as there are reactants in the anode and cathode, and the circuit is closed, secondary batteries continue to produce electrical energy through the electrochemical reactions. Over time, the chemical reactions deplete the reactants in the anode and cathode, which reduces the battery's ability to generate electrical energy.
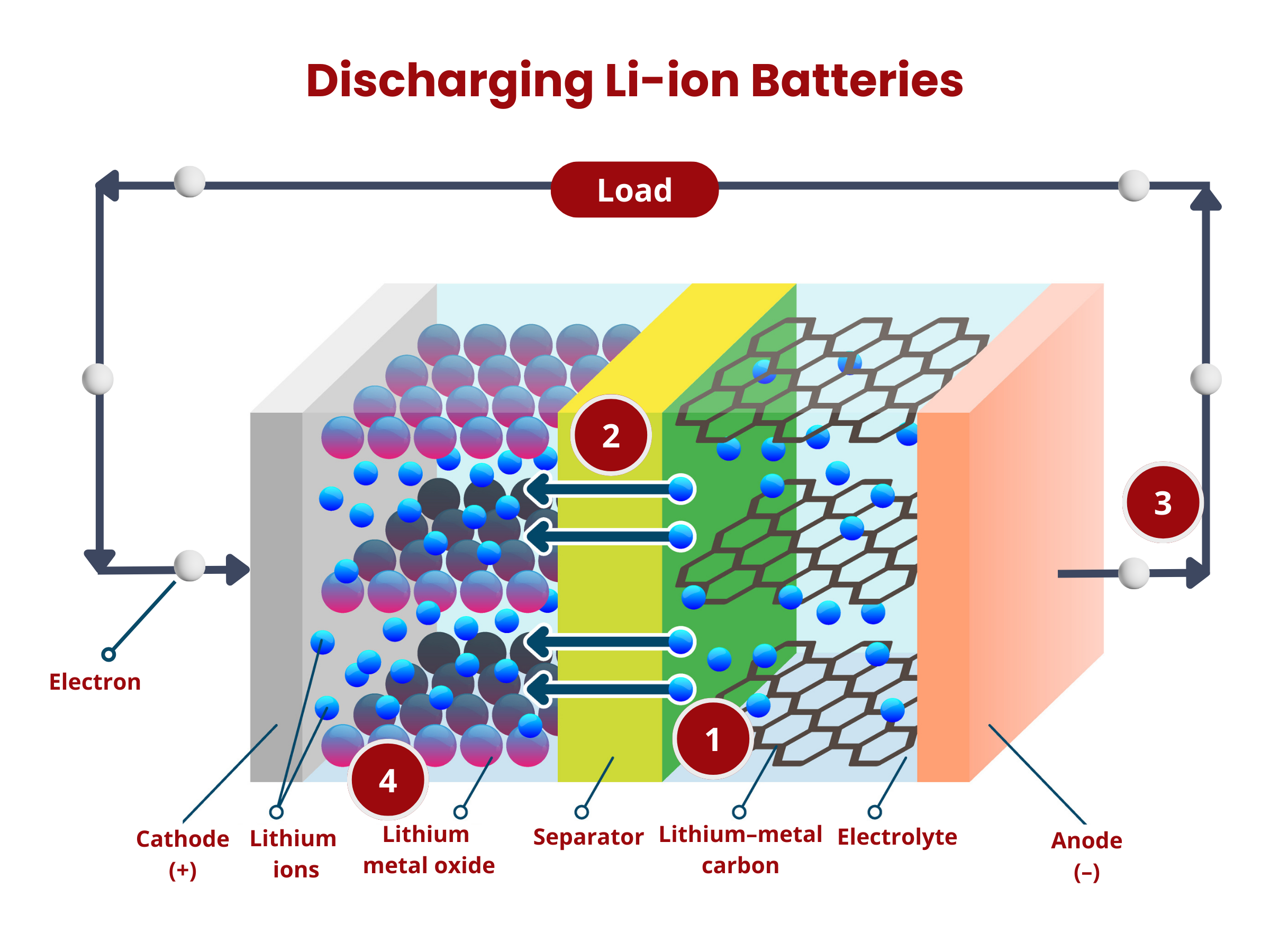
- Oxidation occurs at the anode. The graphite intercalation compound LiC6 is broken down into graphite (C6) and lithium ions, according to the reaction:
LiC6 → C6 + Li+ + e- - The lithium ions move through the electrolyte to the separator until they reach the positive electrode.
- The electrons produced in (1) move from the anode to the cathode through the external circuitry (wiring).
- Reduction occurs at the cathode using the electrons and lithium ions produced in (1). Cobalt oxide (CoO2) reacts with the lithium ions, producing LiCoO2 according to the reaction
CoO2 + Li+ + e- → LiCoO2
- The applied voltage forces the electrons to move from the cathode to the anode. This reverses the reduction reaction during the discharge process, causing the deintercalation of lithium ions from the cathode material:
LiCoO2 → CoO2 + Li+ + e- - The electrons move from the cathode to the anode through the external circuitry (wiring).
- The lithium ions move through the electrolyte to the separator until they reach the negative electrode.
- Intercalation happens at the anode, where lithium ions are inserted back into the anode material (graphite C6), according to the reaction:
C6 + Li+ + e- → LiC6
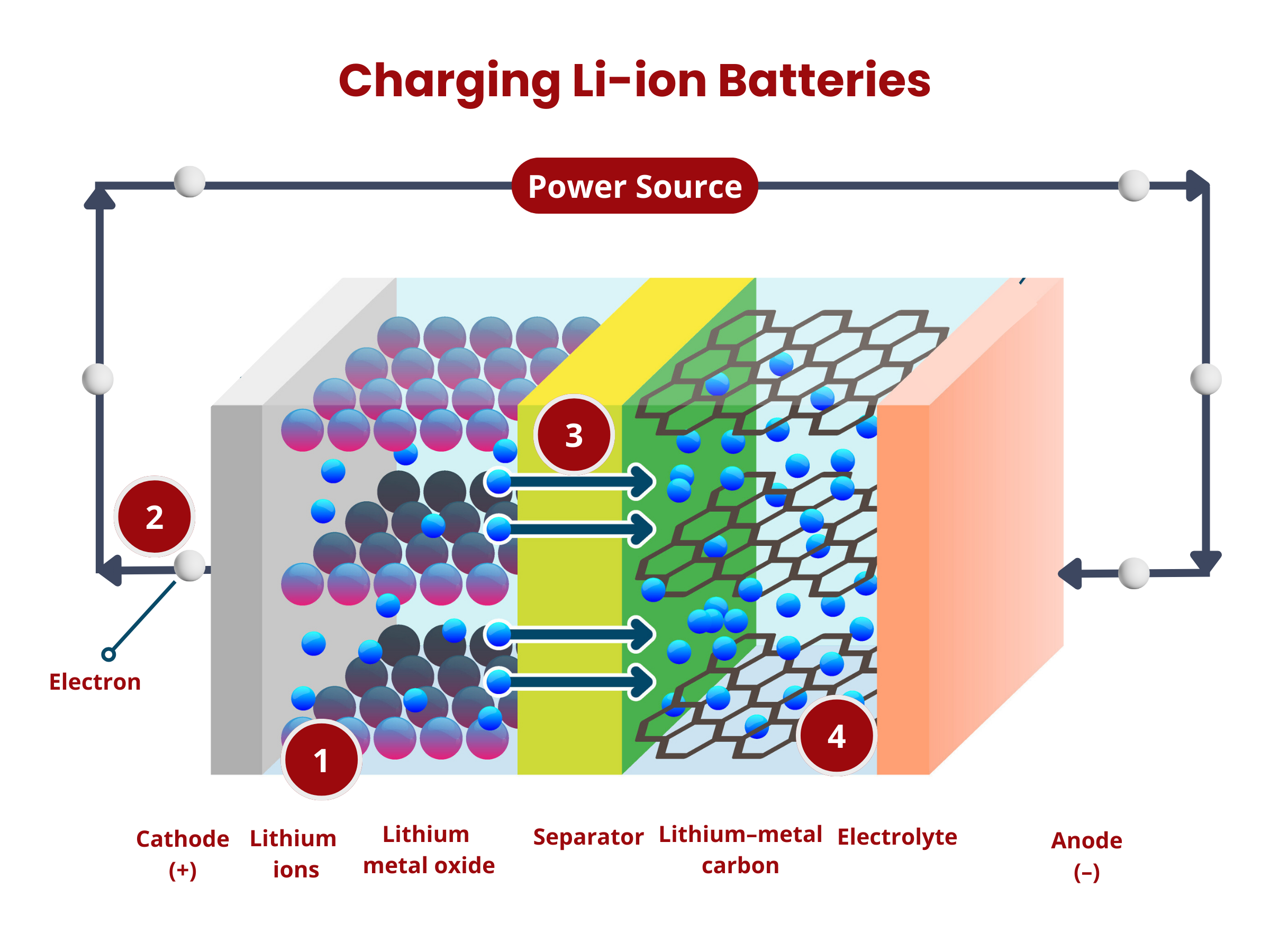