Battery Components
Battery Market Segmentation
The battery market is categorically divided into consumer, automotive, industrial, and special applications, which include aerospace and military sectors.
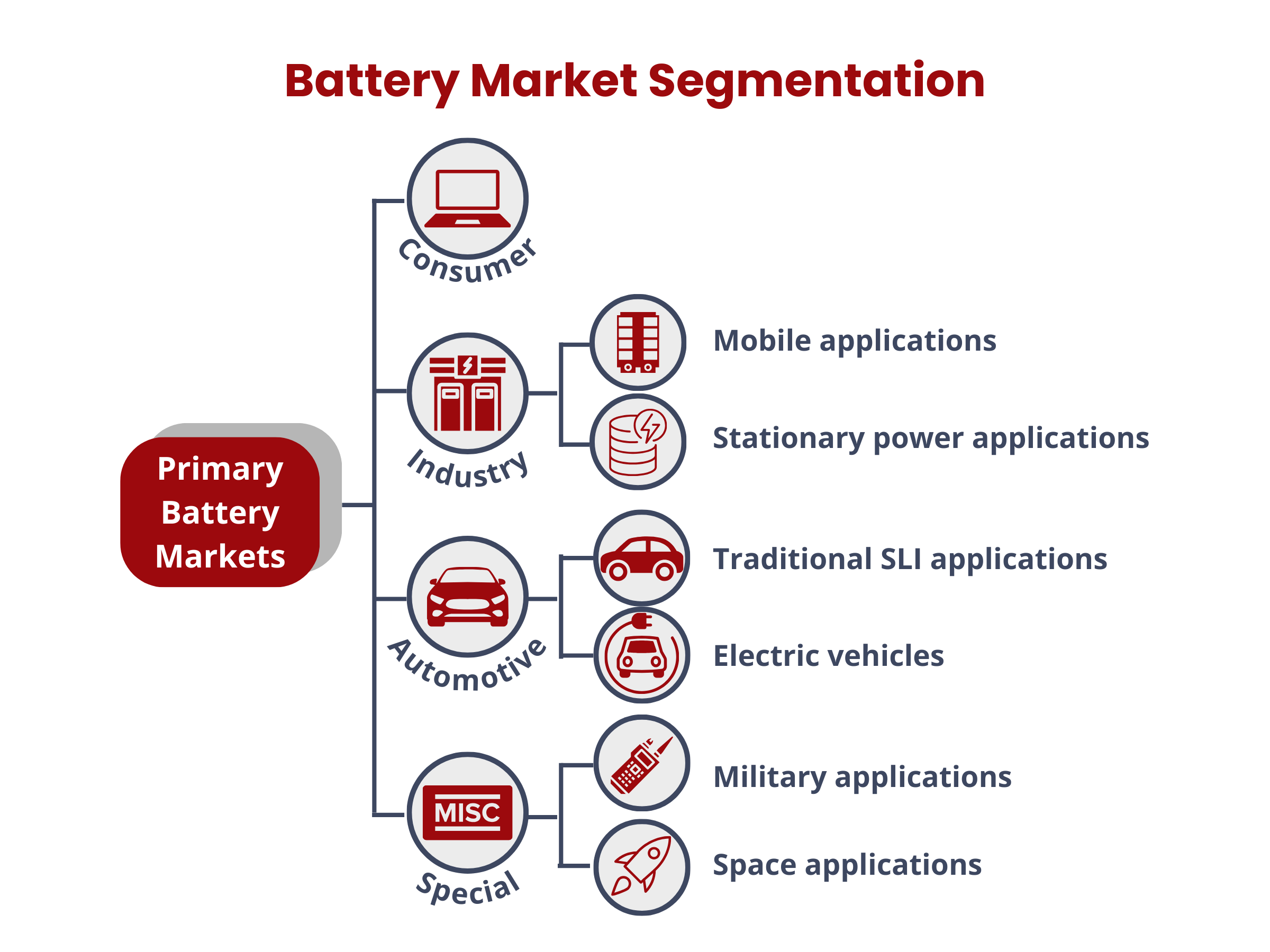
In consumer electronics, lithium-ion batteries have become the major rechargeable power sources due to their high energy density, lightweight nature, and long cycle life. This chemistry is favored for its ability to deliver sustained and reliable performance in devices such as smartphones, laptops, cameras, and portable electronic gadgets. Automotive applications traditionally use batteries for Starting, Lighting, and Ignition (SLI), with lead–acid being the exclusive chemistry employed. Newer automotive systems, such as Hybrid Electric Vehicles (HEV), Plug-in Hybrid Electric Vehicles (PHEV), and Electric Vehicles (EV), employ other battery chemistries. The industrial sector further classifies batteries for mobile and stationary applications. This segmentation underscores the varied and evolving requirements across sectors within the battery industry.
Considering their wide range of applications, electrochemical cells and batteries can also be classified according to their shapes, sizes, and designs. Ensuring optimal, safe, and reliable battery pack design involves a crucial technological step: proper cell containment and packaging.1
Cell components
An electrochemical cell, or simply cell, is the basic electrochemical unit of batteries. In these cells, the conversion of chemical to electrical energy through the reduction and oxidation of electrochemically active materials occurs.
Cells are composed of these four major components: (1) positive and (2) negative electrodes, (3) electrolyte, and (4) separator.2
Electrodes
Battery cells have positive (cathode) and negative (anode) electrodes. During the discharging of secondary battery cells, electrons from an external circuit go to the cathode, where they drive the reduction of the electrochemically active material. On the anode, oxidation occurs, producing the needed electrons for the reduction reaction on the cathode. Both electrodes are composed of two primary components: an active material and a substrate or current collector.
The active material is the component that undergoes reversible chemical reactions during the electrochemical processes of discharging (energy release) and charging (energy storage). In the context of cathode and anode materials in rechargeable batteries, the active material plays a crucial role in the movement of ions and electrons between the electrodes, contributing to the overall energy storage and release capabilities of the battery.
- Cathode active materials (CAM) are commonly metal oxides. However, its specific composition depends on the target electrochemical properties and requirements of the battery chemistry in use. For example, for the commercially dominant lithium-ion batteries, the most common CAMs are lithium cobalt oxide (LiCoO2), lithium manganese oxide (LiMn2O4), lithium iron phosphate (LiFePO4 or LFP), and lithium nickel manganese cobalt oxide (LiNiMnCoO2 or NMC). Similarly, in other battery chemistries like sodium-ion, nickel-metal hydride (NiMH), or lithium-sulfur batteries, different cathode materials are employed. The choice of cathode material is influenced by factors such as energy density, voltage characteristics, cost, and safety considerations.3
- On the other side, anode active materials (AAM) are mainly carbon-based, with graphite being the most widely used due to its high conductivity, affordability, and stable structure. Silicon anodes, while providing higher energy density, face challenges related to volume expansion and shorter cycle life. Some approaches involve 'doping' graphite anodes with a small amount of silicon to enhance performance and energy density.
The active materials are coated onto substrates or current collectors using a slurry, composed of the active material, polymeric binder, and carbon additive. The polymeric binder and carbon additive enhances the adhesion of the active material to the substrate and increases the conductivity of the coating, respectively. Current collectors provide mechanical support to the active materials. In addition, they also provide conductive paths at which the electrons can travel towards the external circuitry.4 Current collectors are equally important cell components as controlling their properties yield significant performance improvements. Reducing their thickness and increasing strength allows the stacking of more active materials in confined spaces, increasing the volumetric energy density of the battery. Meanwhile, improving the adhesion between the active material and CC establishes additional electron pathways, decreasing the internal resistance within the cell. The most commonly used anode and cathode current collectors are Cu and Al foils, respectively. In lithium-ion batteries, Cu foil is stable under the anodic potential (0–1.5 V vs. Li/Li+), whereas Al foil works well under the cathodic potential (3–4.7 V vs. Li/Li+). Interchanging the current collector is not possible because Cu foil undergoes dissolution at potentials > 3.5 V (vs. Li/Li+), and Al makes an alloy with Li at 0.26 V (vs. Li/Li+).
Electrolyte
The electrolyte in a battery facilitates the movement of charged ions between the cathode and anode, enabling the charging and discharging processes. It usually comes in liquid or paste form. The electrolyte's primary function remains consistent across various battery types. It serves as the medium for transporting charged ions, a crucial function in the overall operation of the battery.
Separator
In battery design, a separator, acting as an electrical insulator, prevents direct contact between positive and negative electrodes while facilitating the transfer of ions (ionic conductivity). The electrodes, separator, and electrolyte are assembled within a case or container, with terminals providing electrical connections to the external circuit. For safety, cells may include features like valves or current interrupt devices. Manufacturers have introduced various separator materials, with notable progress occurring in the second half of the 20th century in the chemical industry. Battery separators are categorized based on physical and chemical characteristics, composition, and structure, resulting in types such as nonwoven, microporous, ion-exchange, and nanoporous separators.5
Components of Primary Battery Cells
Battery Chemistry | Anode Material | Cathode Material | Electrolyte | Operating Voltage |
---|---|---|---|---|
Alkaline | Zinc | Manganese dioxide | Alkaline | 1.5 V |
Zinc-carbon | Zinc | Manganese dioxide | Aqueous | 1.5 V |
Lithium | Lithium | Manganese dioxide | Lithium salt in organic solvent | 3 V |
Lithium Iron Phosphate (LiFePO4) | Lithium | Iron phosphate | Organic | 3.2 V |
Lithium Manganese Dioxide (Li–MnO2) | Lithium | Manganese dioxide | Organic | 3 V |
Zinc–silver oxide | Zinc | Silver oxide (Ag2O), C | Alkaline (KOH solution) | 1.55 V |
Zinc–air | Zinc | Oxygen (Air), C | Aqueous (KOH solution) | 1.4 V |
Components of Secondary Battery Cells
Battery Chemistry | Anode Material | Cathode Material | Electrolyte | Operating Voltage | Typical Application |
---|---|---|---|---|---|
Nickel Cadmium (NiCd) | Nickel | Cadmium | Alkaline Potassium Hydroxide | 1.2 V | Portable power tools, backup power |
Nickel Metal Hydride (NiMH) | Nickel | Metal hydride | Alkaline Potassium Hydroxide | 1.2 V | Consumer electronics, hybrid vehicles |
Lead Acid | Lead | Lead dioxide | Sulfuric acid | 2 V (per cell) | Automotive, uninterruptible power supplies |
Lithium Ion | Carbon (usually graphite) | Lithium compound (e.g., LiCoO2) | Organic (typically lithium salt) | 3.6 - 3.7 V (nominal) | Consumer electronics, electric vehicles |
Lithium Sulfur | Lithium | Sulfur | Solid (often polymer) | 2.2 - 2.6 V (nominal) | Aviation, space applications |
Lithium Polymer (Li-Po) | Carbon (usually graphite) | Lithium compound | Gel or polymer (non-liquid) | 3.7 V (nominal) | Portable electronic devices |
Sodium Ion | Carbon | Sodium compounds | Organic or solid-state | Varies | Grid energy storage, renewable integration |
Nickel Hydrogen | Nickel | Hydrogen | Potassium hydroxide | 1.2 V | Satellites, space applications |
Solid-State | Various solid materials | Various solid materials | Solid-state electrolyte | Varies | Evolving technology with potential applications across various sectors |
Battery vs. Cell
Now, a battery is composed of one or more of these electrochemical cells that are electrically connected to achieve the required operating voltage or current. Therefore, the term “battery” can be used to denote a single-cell or multi-cell battery. In addition to cell components, batteries have various elements, such as control and monitoring components, case/container, cell connectors, markings, and supplementary equipment like fuses, communication systems, as well as cooling and venting systems.
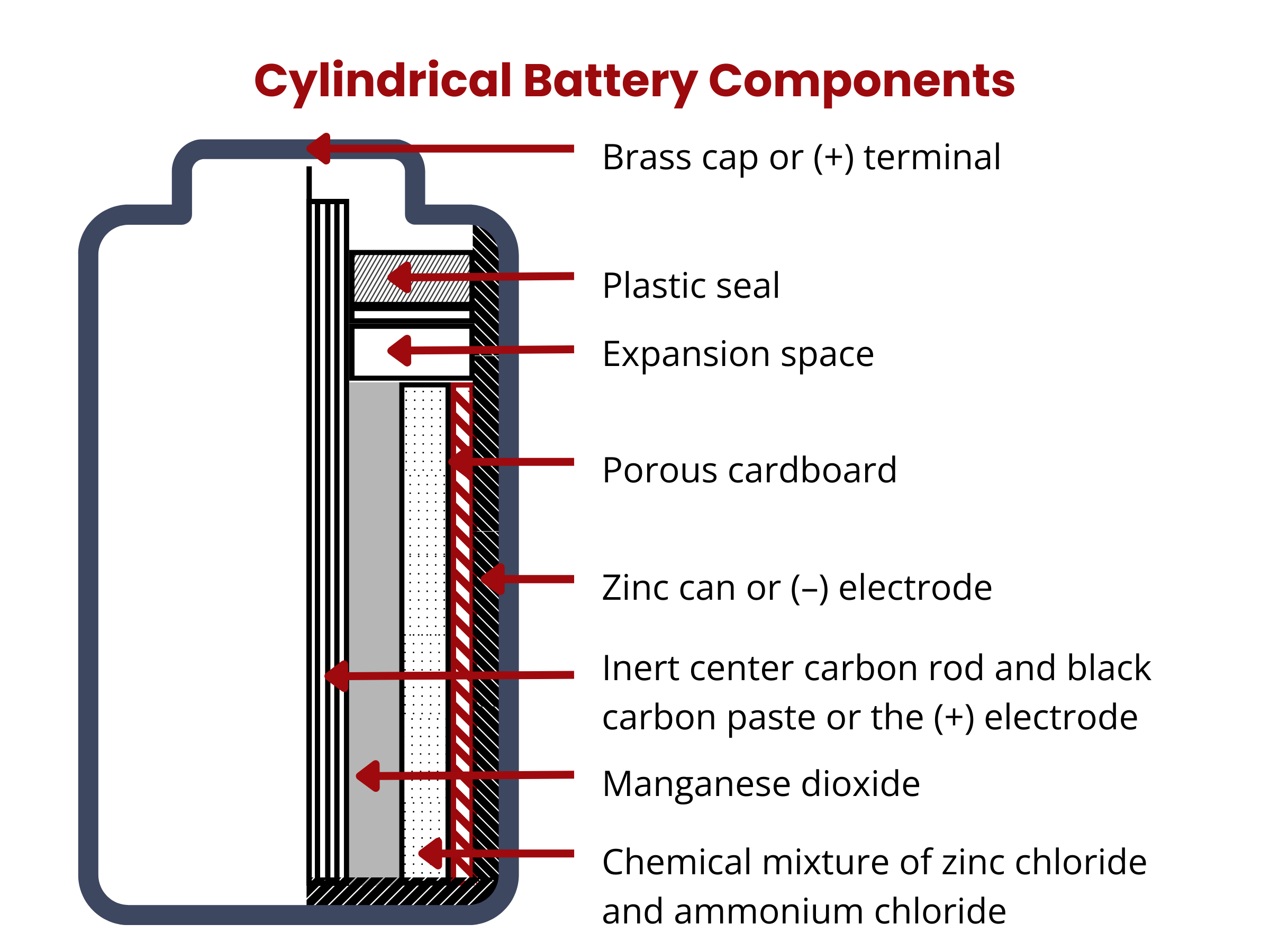
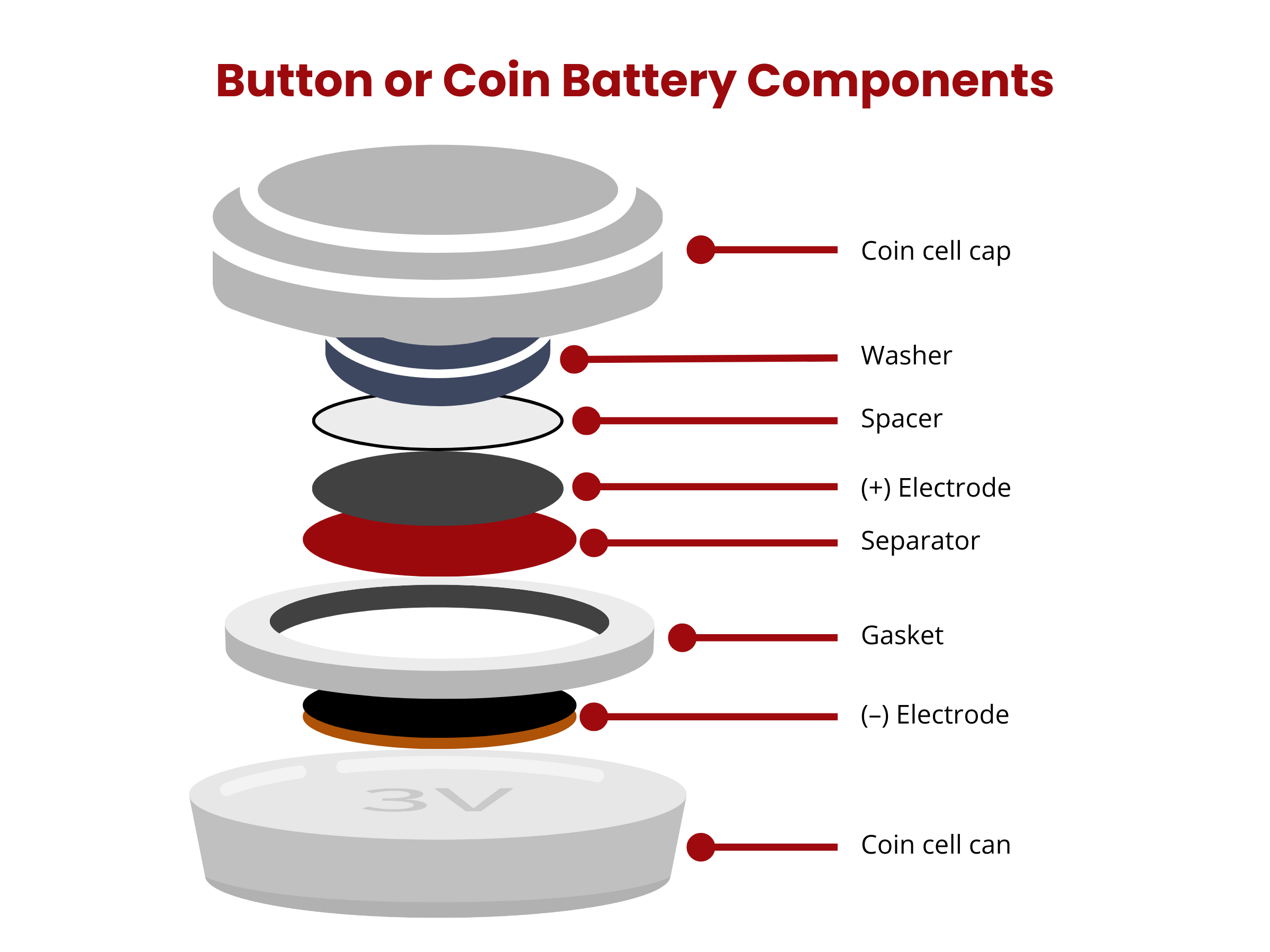
Electric vehicles (EVs) often require more than one battery (or battery module) to achieve the necessary operating voltage and current levels. In such cases, EV battery packs are used. Battery packs are the larger units that house and interconnect multiple modules, providing the overall energy storage for the vehicle, whereas a battery module is a self-contained unit consisting of several individual battery cells. The hierarchy is often as follows: individual cells → modules (batteries) → packs. Learn more information about materials for EV battery packs here.
References
1 Matthias Herrmann; Packaging - Materials review. AIP Conf. Proc. 16 June 2014; 1597 (1): 121–133.
2 Weicker, Phillip. (2014). Systems Approach to Lithium-Ion Battery Management - 2.2 Battery Construction. Artech House.
3 What are battery anode and cathode materials? (2023, May 2). Retrieved from https://www.aquametals.com/recyclopedia/lithium-ion-anode-and-cathode-materials/
4 Hyebin Jeong, Jooyoung Jang, Changshin Jo, A review on current collector coating methods for next-generation batteries, Chemical Engineering Journal. 2022; 446 (1): 136860
5 Boddula, Rajender Inamuddin Pothu, Ramyakrishna Asiri, Abdullah M.. (2020). Rechargeable Batteries - History, Progress, and Applications - 13.3 Classification of Separator in Rechargeable Batteries. John Wiley & Sons.